Now You Know Green Chemistry and Its Application in Textile Industry
Saturday, 2 February 2019
Edit
Green Chemistry and Its Application in Textile Industry
Dr. Asim Kumar Roy Choudhury
Ex-Professor and HOD (Textile),
Govt. College of Engg. & Textile Technology, Serampore - 712201, Hooghly (W.B.), India
E-mail: akrc2008@yahoo.in
Ex-Professor and HOD (Textile),
Govt. College of Engg. & Textile Technology, Serampore - 712201, Hooghly (W.B.), India
E-mail: akrc2008@yahoo.in
Abstract:
Conventional chemical processes based on fossil fuels are unsustainable. Green reactions are sustainable, more efficient (fewer steps, fewer resources, less waste), easier to use (stable under ambient conditions), eco-friendly (non-hazardous solvents and less hazardous waste). They are assessed by twelve principles, the most important being the amount of waste generated. The textile industry is considered as the most ecologically harmful industry in the world. Recently a number of steps have been taken to make textile processing greener. These include use of greener fibre, greener dyes and auxiliaries, greener solvents, eco-friendly, optimised and efficient processing, bio-processing, recycling of textile, water and chemicals and elimination of hazardous chemicals.
Concept of Green Chemistry
Green chemistry looks at pollution prevention on the molecular scale and is an extremely important area of chemistry due to the importance of chemistry in our world today and the implications it can show on our environment. The green chemistry program supports the invention of more environmentally friendly chemical processes which reduce or even eliminate the generation of hazardous substances.
Green chemistry is the chemistry that
- Doesn't hurt nature,
- Reduce or eliminate the use or generation of hazardous substances [1],
- Provides more eco friendly alternative,
- Prevents formation of waste,
- Creates new knowledge based on sustainability i.e. sustainable chemistry [2],
- Takes a life cycle approach to reduce the potential risks throughout the production process. Life Cycle analyses (LCAs) enable a manufacturer to quantify how much energy and raw materials are used, and how much solid, liquid and gaseous waste is generated, at each stage of the product's life (Figure 1).
![]() |
Figure 1 |
The conventional chemical manufacturing processes are unsustainable because:
- Mostly carbon-based products are derived from fossil fuels, petroleum and coal which have limited supply.
- Large amounts of waste increasing burden on the environment.
In developing countries, although there is growing awareness about the ill effects of pollution, promotion of continual introduction of environmentally friendly products a methodologies in the chemical industry needs to be developed further. Usage of non-conventional technologies is highly popular in India. First in this list is the usage of microwaves. Further, the microwave chemists are turning their attention toward microwave-assisted dry-media reactions in order to minimize solvent usage, an added advantage to already established microwave chemistry. In addition to microwave-assisted reactions, ultrasonic and photochemical reactions are also used as non-conventional reaction technology [3]. The strict application of the Euro norms and the drive for switchover to Compressed Natural Gas (CNG) as an alternative fuel are some of the examples of the endeavors made by India towards creating a greater eco-friendly environment.
Principles of Green Chemistry
Twelve principles of green chemistry are:
The easiest way to assess how green a chemical process is to measure the amount of waste generated. E-factor (Environmental acceptability) measures the ratio of the mass of waste to that of the product [5]. All processes should aim for the lowest possible E-factor - for truly green processes, the E-factor should be zero. Large-scale manufacturing units for bulk chemicals may generate large amount of waste, but their E-factors may be smaller than those of small-scale units as the E-factor depends on the quantity of waste in relation to total production.
Twelve principles of green chemistry are:
- Prevent waste: Design chemical syntheses to prevent waste, thereby eliminate/minimise waste treatment processes. It is better to prevent waste than to treat or clean up waste after it is formed.
- Maintain atom economy: There should be few, if any, wasted atoms.
- Use safe chemical synthesis methods
- Use low toxic products: Use fully effective but safe or non-toxic chemicals and products.
- Choice energy efficient processes: Prefer ambient temperature and pressure reactions.
- Use renewable feedstock: Use non-depleting renewable agricultural products or the wastes of other processes and not products derived from fossil fuels.
- Omit derivation steps: Follow least number of sequential chemical steps, and choose direct reactions.
- Catalysis: Catalytic reactions generate minimum waste – its little amount can carry out a single reaction many times. Gold is an outstanding catalyst for oxidation processes [4].
- Safer solvents and auxiliaries: Use aqueous or other safe media.
- Degradation of chemical products: Choose chemicals degradable to harmless substances.
- Real time analysis: Minimise/eliminate by-products by real-time monitoring and control.
- Safety: Assure minimum chemical accidents (e.g. explosions, fires and harmful releases).
The easiest way to assess how green a chemical process is to measure the amount of waste generated. E-factor (Environmental acceptability) measures the ratio of the mass of waste to that of the product [5]. All processes should aim for the lowest possible E-factor - for truly green processes, the E-factor should be zero. Large-scale manufacturing units for bulk chemicals may generate large amount of waste, but their E-factors may be smaller than those of small-scale units as the E-factor depends on the quantity of waste in relation to total production.
Misconceptions
Some misconceptions about green chemistry [9] are:
Some misconceptions about green chemistry [9] are:
- Cost Benefit: Green chemistry truly allows for increased profits by saving reagents, solvents, energy, waste disposal costs, personnel costs, and increasing production.
- Perfection of the Systems: A perfectly green process may not be so green if it hasn't been applied in the right situations.
- Fields of Application: The application of green chemistry is not restricted. It is applicable to various industrial sectors since all industrial processes involve one or more of the following basics: raw materials, chemical reactions, solvents, and separation/purifications.
- Longevity: Green chemistry often remains unchanged for long periods of time.
- Overall Performance: Traditional purification and separation methods both generate large amounts of acid, base, and solvent wastes, and are often energy intensive. New separation techniques such as carbon dioxide extraction, phase separation, evaporation, membrane separation, and reforming by-products into new products minimise waste generation.
Textile industry and pollution
The textile industry is considered as the most ecologically harmful industry in the world. The utilization of rayon for clothing affects fast depleting forests. Petroleum-based synthetic fibre and the dyes are not sustainable and not biodegradable [13]. Cotton cultivation requires large quantities of synthetic fertilizers and pesticides/herbicides. Presently the conventional cotton crops occupy 3% of the world cultivated areas. Nevertheless, it represents 25 % of pesticides and 10 % of insecticides bought in the world.
Water Consumption
Estimated total water used in wet processing of cellulosic fibres is 2.96 trillion litres considering water consumption of 100 l/kg of material. If we can reduce water consumption by ¾ (i.e. 25 l/kg), the saved water can provide drinking water for 2.34 billion people (assuming consumption of 2.6 litres per capita per day).
Water can be saved in dyeing in the following ways:
The important environmental concerns related to textile wet processing are:
Adsorbable organic halogens (AOX)
It is a measured value for organically bound chlorine, bromine and iodine in a given substance. The AOX consent limit is likely to be as low as 2 ppm from a German drinking water directive (DIN 38409414, 1987) [15] and as such compounds having high AOX values are to be used carefully, a few such products used in the textile industry are:
Some of the toxic and harmful substances used in textile industries and their eco-friendly substitutes are listed in Table 1 [16]:
The textile industry is considered as the most ecologically harmful industry in the world. The utilization of rayon for clothing affects fast depleting forests. Petroleum-based synthetic fibre and the dyes are not sustainable and not biodegradable [13]. Cotton cultivation requires large quantities of synthetic fertilizers and pesticides/herbicides. Presently the conventional cotton crops occupy 3% of the world cultivated areas. Nevertheless, it represents 25 % of pesticides and 10 % of insecticides bought in the world.
Water Consumption
Estimated total water used in wet processing of cellulosic fibres is 2.96 trillion litres considering water consumption of 100 l/kg of material. If we can reduce water consumption by ¾ (i.e. 25 l/kg), the saved water can provide drinking water for 2.34 billion people (assuming consumption of 2.6 litres per capita per day).
Water can be saved in dyeing in the following ways:
- Reuse dyehouse water
- Reduce reprocessing
- Optimise rinsing and soaping processes
- Reduce Liquor ratio
The important environmental concerns related to textile wet processing are:
- Chemical intensive wet processing– scouring, bleaching, mercerizing, dyeing, printing etc.
- Use of heavy metals – iron, copper, lead etc, found in dyestuffs auxiliaries, binders etc.
- Residual dyestuffs due to poor fixation of dyes and chemicals in effluent water.
- PVC and phthalates used in plastisol printing paste.
- Formaldehyde found in dispersing agents, resins, printing paste and colorant fixatives.
- Dye effluent-wastewater issue.
Adsorbable organic halogens (AOX)
It is a measured value for organically bound chlorine, bromine and iodine in a given substance. The AOX consent limit is likely to be as low as 2 ppm from a German drinking water directive (DIN 38409414, 1987) [15] and as such compounds having high AOX values are to be used carefully, a few such products used in the textile industry are:
- Chlorine-containing bleaching agent.
- Shrink-proofing of wool with chlorine, the promising alternate being permonosulphuric acid.
- Insect-proofing agent for wool.
- Some types of carriers used in dyeing with disperse dyes.
- Certain chromophores.
- Some classes of reactive dyes.
Some of the toxic and harmful substances used in textile industries and their eco-friendly substitutes are listed in Table 1 [16]:
Table 1. Some Harmful Textile Chemicals and Their Eco-friendly Substitutes | ||
Existing Chemicals | Uses | Proposed substitutes |
Polyvinyl alcohol (PVA) | Yarn size | Potato starch or carboxymethylcellulose (CMC) |
Pentachlorophenol, formaldehyde | Size preservative | Sodium silicofluride |
Carbon tetrachloride (CTC) | stain removers |
|
Calcium and sodium hypochlorite | Bleaching | Hydrogen peroxide, ozone at cold |
Sodium silicate, phosphorous-based compounds | Peroxide stabiliser | Nitrogenous stabilisers |
Nonyl phenyl ethylene oxide adducts (APEO) | Detergent, emulsifier | Fatty alcohol ethylene oxide adducts, alkylpolyglycosides |
Synthetic non-biodegradable surfactants | Various purposes | Sustainable and highly biodegradable surfactants from dextrins |
Synthetic non-biodegradable surfactants + solvent | Coatings and degreasing | ‘solvosurfactants’ acting both solvent and surfactant, derived from glycerol (bio diesel) |
Dichloro and trichloro benzene | Carriers in dyeing | Butyl benzoate, benzoic acid |
Kerosene | Pigment printing | Water-based thickeners |
Formaldehyde | Finishing, dye fixing | Polycarboxylic acid, non-formaldehyde products |
Sodium dichromate | Oxidation in dyeing | Hydrogen peroxide |
Silicones and amino-silicones + APEO emulsifier | Softener | Eco-friendly softeners, wax emulsions |
Functional synthetic finish | Finishing | Bees wax, aloe vera and Vitamin A (Hazardous Substance Research Centers/ South & Southwest Outreach Program, “Environmental Hazards of the Textile Industry,” Environmental Update #24, Business Week, June 5, 2005; http://www.oecotextiles.com/) |
Green Chemistry in Textile Industry
In paper and textile industries, efforts are being made to develop new greener methods, which result in reduction in energy, water usage, time in textile processing. Some examples of green approaches in various textile related industries are as follows:
Greener Fibres
Organic cotton is generally understood as cotton, from non-genetically-modified plants, that is certified to be grown without the use of any synthetic agricultural chemicals such as fertilizers or pesticides. The farmers undertake not to use chemicals, and to recycle as much as possible the waste stemming from their activity.
Lyocell fibres are produced by regenerating cellulose in an organic solvent, N-methylmorpholine-N-oxide (NMMO) hydrate. Non-toxic, biodegradable NMMO solvent used is almost completely recycled [17]. The fibre is significantly more sustainable than oil-derived synthetic fibres and natural fibres such as cotton (need pesticides and fertilisers to grow). Land required is less in case of the eucalyptus trees, from which lyocell is made [18].
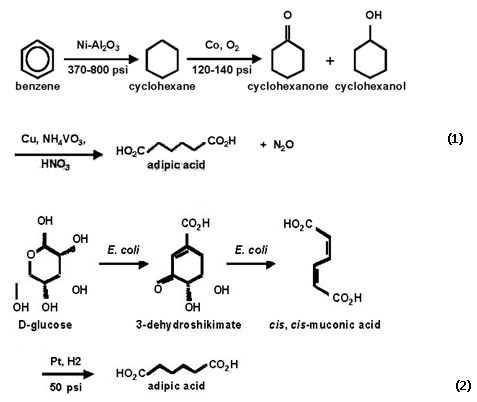
Polyurethane polymers are presently produced from toxic diisocyanate. A series of polyurethanes based on bis-carbamate diols are synthesised using the Candida antarctica lipase B as catalyst.
The use of biopolymers – plastics made from corn, sugar, starch and other renewable raw materials – has exploded in recent years. The chemical structures of a few biopolymers are shown in Figure 2.
![]() |
Figure 2: Chemical Structures of some biopolymers (a) polylactic acid (b) Poly-(R)-3-hydroxybutyrate (P3HB) (c) polycaprolactone (Source: http://en.wikipedia.org). |
The route of manufacture is as follows:
Corn → starch → unrefined dextrose → fermentation → lactic acid → monomer production → lactide → polymer (PLA) production → polymer modification → fibre, film, plastic, bottle etc. (3)
Clariant announced the development of Biodegradable RENOL®-natur colorant from sustainable, mainly vegetable resources for use with these new-age plastics. [19].
Henry Ford first used soy plastic to construct various car parts. A new composite materials has been derived from soy flour and flax resin cross-linked with glutaraldehyde, derivable from bio-feedstocks. Materials produced have good mechanical properties and may be used for indoor applications [20]
Recycled Textiles
Because textiles are nearly 100% recyclable, nothing in textile and apparel industry should be wasted. The textile recycling industry is one of the oldest and most established recycling industries in the world. Textile recycling materials may be pre-consumer or post consumer (i.e. used garments or articles). The sorting categories of textile recycling by volume is represented by a pyramid structure, the base of which consists of used cloth market (48%), followed by conversion to value added new materials (29%), cut into wiping and polishing cloths (17%), landfill and incineration for energy (<7%). The peak of the pyramid is represented by ‘Diamonds’ (1-2%) which have high value for antique quality or for other reason. [21].
Polyester fibre is one of the most non-biodegradable polymers which create environmental problems. Major revolution happened in 1993 when Wellman Inc. introduced the first polyester textile fibre made from post consumer PET packaging: Fortrel® EcoSpun®. There are two broad types of recycled polyester namely:
If the carpet fibres are made of polypropylene and they’re held together with a polypropylene Licocene back-coating, the product can be reused simply by melting [19].
Greener Dye and auxiliaries
The greener approaches are:
By reacting dextrins with fatty acids and their derivatives, new sustainable and biodegradable surfactants have been formed. They have highly desirable physical properties including low foaming, good wetting and whitening ability, as well as excellent biodegradability [24].
Queste reported [25] that the researchers in France and Germany have jointly developed a new class of so-called ‘solvosurfactants’ (which exhibit the properties of both solvent and surfactant and are commonly used in applications such as coatings and degreasing, as well as perfumery and inks) that are derived from glycerol, a renewable material from bio diesel.
Greener Preparation and Dyeing
Bio-processing can simply be defined as the application of living organisms and their components to industrial products and processes, which are mainly based on enzymes.
a) Improvement in the existing dyeing processes
Formaldehyde-free Finishing Agents
The most widely used crosslinking agents in DP finishes, N-methylol agents or N-methylolamides fall in the category of formaldehyde reactants [35]. The release of formaldehyde vapours is a problem with those agents. It depends on the reactant types, the catalyst types, the condition of the treated fabrics, and the additives in the impregnating bath and most importantly the time and temperature of cure [36].
Corn → starch → unrefined dextrose → fermentation → lactic acid → monomer production → lactide → polymer (PLA) production → polymer modification → fibre, film, plastic, bottle etc. (3)
Clariant announced the development of Biodegradable RENOL®-natur colorant from sustainable, mainly vegetable resources for use with these new-age plastics. [19].
Henry Ford first used soy plastic to construct various car parts. A new composite materials has been derived from soy flour and flax resin cross-linked with glutaraldehyde, derivable from bio-feedstocks. Materials produced have good mechanical properties and may be used for indoor applications [20]
Recycled Textiles
Because textiles are nearly 100% recyclable, nothing in textile and apparel industry should be wasted. The textile recycling industry is one of the oldest and most established recycling industries in the world. Textile recycling materials may be pre-consumer or post consumer (i.e. used garments or articles). The sorting categories of textile recycling by volume is represented by a pyramid structure, the base of which consists of used cloth market (48%), followed by conversion to value added new materials (29%), cut into wiping and polishing cloths (17%), landfill and incineration for energy (<7%). The peak of the pyramid is represented by ‘Diamonds’ (1-2%) which have high value for antique quality or for other reason. [21].
Polyester fibre is one of the most non-biodegradable polymers which create environmental problems. Major revolution happened in 1993 when Wellman Inc. introduced the first polyester textile fibre made from post consumer PET packaging: Fortrel® EcoSpun®. There are two broad types of recycled polyester namely:
- Simply melted and re-extruded into fibres and
- A multi-stage de-polymerisation and re-polymerisation to produce better quality yarn.
If the carpet fibres are made of polypropylene and they’re held together with a polypropylene Licocene back-coating, the product can be reused simply by melting [19].
Greener Dye and auxiliaries
The greener approaches are:
- Elimination of harmful azo dyestuffs
- Alternative synthesis for eco-friendly products.
- Search for sustainable source such as natural dyes. They, in general, have poor to moderate light fastness. It was found that the natural additives Vitamin C (ascorbic acid) and gallic acid (found in stomach, tea leaves, oak bark and many other plants) were most effective in reducing the rate of fading in madder, weld and woad dyed cotton [23].
By reacting dextrins with fatty acids and their derivatives, new sustainable and biodegradable surfactants have been formed. They have highly desirable physical properties including low foaming, good wetting and whitening ability, as well as excellent biodegradability [24].
Queste reported [25] that the researchers in France and Germany have jointly developed a new class of so-called ‘solvosurfactants’ (which exhibit the properties of both solvent and surfactant and are commonly used in applications such as coatings and degreasing, as well as perfumery and inks) that are derived from glycerol, a renewable material from bio diesel.
Greener Preparation and Dyeing
Bio-processing can simply be defined as the application of living organisms and their components to industrial products and processes, which are mainly based on enzymes.
Related:
The application of enzymes in various stages of textile processing may be listed as follows [26]:
- Desizing: amylase, lipase.
- Scouring: pectinase, cellulase.
- Bleaching: oxidoreductase. xylanase.
- Dyeing: oxidoreductase.
- Finishing: cellulase, oxidoreductase, lipase.
- Composting (biodegradation of textile wastes): cellulase, protease, nylonase, polyesterase.
- Delignification, decolourisation of dyes: laccases [27]
- Purification of cellulose by extraction by carbon dioxide and ionic liquids,
- High temperature water extraction of lignin,
- Substitution of chlorine bleaching with non-polluting oxidants,
- Carbon dioxide-based dry cleaning.
- Elimination of ozone-depleting chemicals such as carbon tetrachloride (stain remover).
a) Improvement in the existing dyeing processes
- Optimise processes (to reduce time and energy consumption)
- Reduce consumption of water, electrical power, steam consumption
- Optimise dye/chemical costs
- Eliminate reprocessing and shade correction
- Sulphur dyeing: substitution of hazardous sodium sulphide with sustainable, nontoxic, biodegradable, cost-effective reducing sugars [28].
- Reactive dyeing: treatment of cellulose with cationic, nucleophilic polymers enables dyeing at neutral pH without electrolyte addition - 3-chloro-2-hydroxypropyl-trimethylammonium chloride (CHTAC) [29], Copolymer of diallyldimethylammonium chloride and 3-aminoprop-1-ene and copolymer of 4-vinylpyridine quaternised with 1-amino-2-chloroethane [30].
- In chemical-free denim processing [31], laser technology is used to burn away the surface of the dyed denim fabric or a pair of jeans on a mannequin to replicate an authentic worn look. The laser system is very quick and a pair of jeans can take as little as 15 seconds to process.
- Right-First-Time dyeing: It is also termed as ‘no addition’ dyeing or ‘blind dyeing’. Elimination of the inspection stage made a significant saving [32]. Twenty factors which must be monitored or controlled to achieve RFT processing in the dyeing process have been identified [33].
- About 90% dye fixation on batchwise cellulose dyeing with polyfunctional dyes.
- Cold pad-batch dyeing, rapid dyeing techniques and better machine design.
- Economic continuous dyeing methods.
- Supercritical carbon dioxide dyeing (Figure 3)
![]() |
Figure 3. Supercritical State of CO2 |
The most widely used crosslinking agents in DP finishes, N-methylol agents or N-methylolamides fall in the category of formaldehyde reactants [35]. The release of formaldehyde vapours is a problem with those agents. It depends on the reactant types, the catalyst types, the condition of the treated fabrics, and the additives in the impregnating bath and most importantly the time and temperature of cure [36].
The Occupational Safety and Health Administration (OSHA) have set the upper limit for formaldehyde in air at 0.75 parts per million averaged over an eight-hour work shift [37]. Formaldehyde is a carcinogen to animals [36]. Some formaldehyde-free DP finishes are:
An interesting development in the field of flame retardancy is the use of polymer nanocomposites as a substitute of toxic brominated flame retardants (BFR). Nanocomposites may be described as two-phase materials, consisting of a dispersion of appropriate filler (on a nanometre scale) through a polymer matrix. In the case of polymer-layered silicate (clay) nanocomposites, only a very small amount of filler (2-10 weight-%) is required for the material to be flame-retardant [39].
Automation
Pollution reduction is possible through automation in textile dyeing and printing. The steps are:
For improvement in the quality: steps (a) and (c) must be adopted
For man-power savings: steps (a) and (b) are to be followed
For cost reduction: step (d) is to be adopted.
For better customers’ service (RFT production, quick response and just-on-time delivery): steps. (a) to (e) (full automation).
- Cyclic addition of glyoxal with NN/dimethyl urea, namely DHDMI (1,3 dimethyl-4,5-dihydroxyethyleneurea)
- Polycarboxylic acids (PCA) - their main drawback is loss of tensile strength due to acid-catalysed cellulose chain cleavage. The most important PCA reactants are butanetetracarboxylic acid (BTCA) and citric acid (CA) [38]. BTCA, in the presence of sodium hypophosphite, provides the same level of durable press performance as conventional DMDHEU reactant, but it is quite costly [37].
An interesting development in the field of flame retardancy is the use of polymer nanocomposites as a substitute of toxic brominated flame retardants (BFR). Nanocomposites may be described as two-phase materials, consisting of a dispersion of appropriate filler (on a nanometre scale) through a polymer matrix. In the case of polymer-layered silicate (clay) nanocomposites, only a very small amount of filler (2-10 weight-%) is required for the material to be flame-retardant [39].
Automation
Pollution reduction is possible through automation in textile dyeing and printing. The steps are:
- Process control - 10-30% saving in water and energy as well as 5-15% saving in dyes and chemicals.
- Auto-dispensing - 5-10% savings in dyes, pigments and chemicals.
- Computer-controlled weighing and stock-taking - 10-15% savings in dyes, pigments and chemicals.
- Colour measurement and matching - significant improvement in quality and 30-40% savings of dyes and pigments.
- Central computer (network), computerised management system.
For improvement in the quality: steps (a) and (c) must be adopted
For man-power savings: steps (a) and (b) are to be followed
For cost reduction: step (d) is to be adopted.
For better customers’ service (RFT production, quick response and just-on-time delivery): steps. (a) to (e) (full automation).
Eco-legislation
REACH [40] is a new European Community Regulation on chemicals and their safe use. It is a new regulatory framework proposed by the EC on October 29, 2003. It is a single, coherent system for new and existing chemicals with the following three new elements:
Green Chemistry provides a technical solution to many environmental problems. It is effective due to design stage efforts, starting at the molecular level lets one to design out the hazardous properties and to design in environmentally appropriate features.
The 21st century philosophy for textile dyeing is follows:
The textile industry is one of the highly revenue generating industries in India and many other developing countries. Pollution created by textile industries is a big problem for them. Modern technologies like microbial decolorization and degradation are slowly being adopted for the treatment of polluted water. There is an increasing need of exploring biodiversity for natural dyes and developing eco-friendly methodology for synthetic dyes and dyeing. All these require more funding in the R&D of respective fields and greater interaction and coordination between industry, academia, and government [43].
References
REACH [40] is a new European Community Regulation on chemicals and their safe use. It is a new regulatory framework proposed by the EC on October 29, 2003. It is a single, coherent system for new and existing chemicals with the following three new elements:
- Registration (30,000 substances traded in EU; 100,000 on EINECS)
- Evaluation (5,000 substances)
- Authorisation (1,350 substances) of Chemical Substances
- To improve the protection of human health and the environment
- To increase the competitiveness of the EU chemicals industry
- To increase transparency
- To achieve integration with global efforts
- To conform with EU international obligations under the WTO
- To promote non-animal testing.
Green Chemistry provides a technical solution to many environmental problems. It is effective due to design stage efforts, starting at the molecular level lets one to design out the hazardous properties and to design in environmentally appropriate features.
The 21st century philosophy for textile dyeing is follows:
- Minimum human/operator intervention,
- Process steps optimised for utility consumption,
- Decisions made strategically not on a daily routine basis,
- Processes devised and selected to produce the correct shade and quality as an expectation not just an intention,
- Digital shade passing and colour communication,
- Profits are made by doing it right - not just by doing it cheaply [41].
- Become carbon neutral,
- Send no waste to landfill,
- Develop sustainable sourcing routes,
- Set new standards in ethical trading,
- Help customers and employees to live a healthier lifestyle.
The textile industry is one of the highly revenue generating industries in India and many other developing countries. Pollution created by textile industries is a big problem for them. Modern technologies like microbial decolorization and degradation are slowly being adopted for the treatment of polluted water. There is an increasing need of exploring biodiversity for natural dyes and developing eco-friendly methodology for synthetic dyes and dyeing. All these require more funding in the R&D of respective fields and greater interaction and coordination between industry, academia, and government [43].
References
- P. Anastas and J. C. Warner, Green Chemistry, Theory and Practice, Oxford University Press, New York, 1998.
- www.epa.gov/ greenchemistry/
- M. Kidwai, Green chemistry in India, Pure Appl. Chem., Vol. 73, No. 8, pp. 1261–1263 (2001).
- Graham J. Hutchings, Green Chemistry has a Golden Future, Europacat7, (August) Cardiff University, UK) (2005).
- Martyn Poliakoff and Pete Licence, Nature, 450 (6) December (2007).
- R. A. Sheldon, (2005). Green Chem., 7, 267 (2005).
- D. C. Rideout and R. Breslow, J. Am. Chem. Soc., 102, 7816 (1980).
- Chao-Jun Li, Green Chem., 10, 151–152, (2008). DOI: 10.1039/b800137p.
- Chao-Jun Li, Canadian Chemical News, April , (2004).
- Ryoji Noyori, Chemical Communications (Royal Society of Chemistry) (14), 1807 – 1811 (2005).
- E. Bach, E Cleve, and E. Schollmeyer, Rev. Prog.Color. 32, 88 (2002).
- M. van der Kraan, Process and Equipment Development for Textile Dyeing in Supercritical Carbon Dioxide, Ph. D thesis at Technische Universiteit, Delft and J. Supercrit. Fluids 34, 99 (2005).
- Z Khatri and Khan Muhammad Brohi, Environmental Friendly Textiles - a road to sustainability, www.Knol.google.com.
- H. Zollinger, Color Chemistry, Synthesis, Properties of Organic Dyes and Pigments, 2nd ed., VCH Publishers, New York, 92 (1987).
- B.M. Müller, Rev. Prog. Coloration, 22, 14-21 (1992).
- A.K. Roy Choudhury Textile Preparation and Dyeing, Science Publishers (Enfield, NH: USA), 2006.
- W Albrecht, M Reintjes and B Wulfhorst, Chem. Fibers Int., 47, 298 (1997)
- P White, M Hayhurst, J Taylor and A Slater, Biodegradable and Sustainable Fibres. (Ed.) R S Blackburn (Cambridge: Woodhead), 157, 2005.
- Walking the talk: Clariant products provide ‘green’ chemistry successes, News Release, www.clariant.com, August 19 (2008).
- S. Chabba et al., Green Chemistry, 7, 576 (2005).
- J. M. Hawley Textile recycling: A system perspective, in Recycling in Textiles, Y Yong (eds), (Cambridge: Woodhead), (2006).
- Dyeing Recycled Pet, www.ecotextile.com.
- D. Cristea & G. Vilarem, Dyes and Pigments, 71, 39 (2006).
- H. J. Wang and K-M. Chen, J. Applied Polymer Science, 98, 711 (2005).
- S. Queste et al., Green Chem., 8, 822 (2006).
- I. Holmes. Colourage Annual, 41-56 (1998)
- K. Li et al., Appl. Environ. Microbiol. 65, 2654–2660, (1999).
- Richard S. Blackburn and Anna Harvey, Environ. Sci. Technol., 38 (14), pp 4034–4039 (2004).
- M Hashem, P Hauser and B Smith, Text. Res. J., 73 , 762 (2003).
- Richard S. Blackburn, Stephen M. Burkinshaw, Journal of Applied Polymer Science, Vol. 89, 1026–1031 (2003).
- Chemical-free denim processing, www.ecotextile.com.
- J Park and J Shore Color. Tech., 125, 133-140 (2009).
- Park and J Shore, Practical Dyeing (Bradford: SDC), 2004.
- T.L. Dawson, Color. Tech., 124, 67-78 (2008).
- H. Peterson, Review of Progress in Coloration and Related Topics, 17, 7-22 (1987).
- T. F. Cooke & H. D. Weigmann, Textile Chemist and Colorist, 14, 100-106.& 136-144 (1982).
- B. A. K. Andrews, Textile Chemist and Colorist, 22, 63-67. (1990).
- C. M.Welch, Textile Research Journal, 58, 480-486 (1988).
- www.eupen.com/highlights/publications.html.
- www.fibre2fashion.com.
- A C Welham, www.dyehousedoctor.com, Textile Dyeing in the Age of Aquarius.
- Mark Sumner, (2009). Plan A & Eco Dyehouses, Part I, (Bradford: Society of Dyers and Colourists).
- A.K. Roy Choudhury, Green Chemistry and the Textile industry, Textile Progress, Volume 45, Issue 1, 2013 (Textile Institute, UK). http://www.tandfonline.com/doi/full/10.1080/00405167.2013.807601
Sumber http://textilelearner.blogspot.com